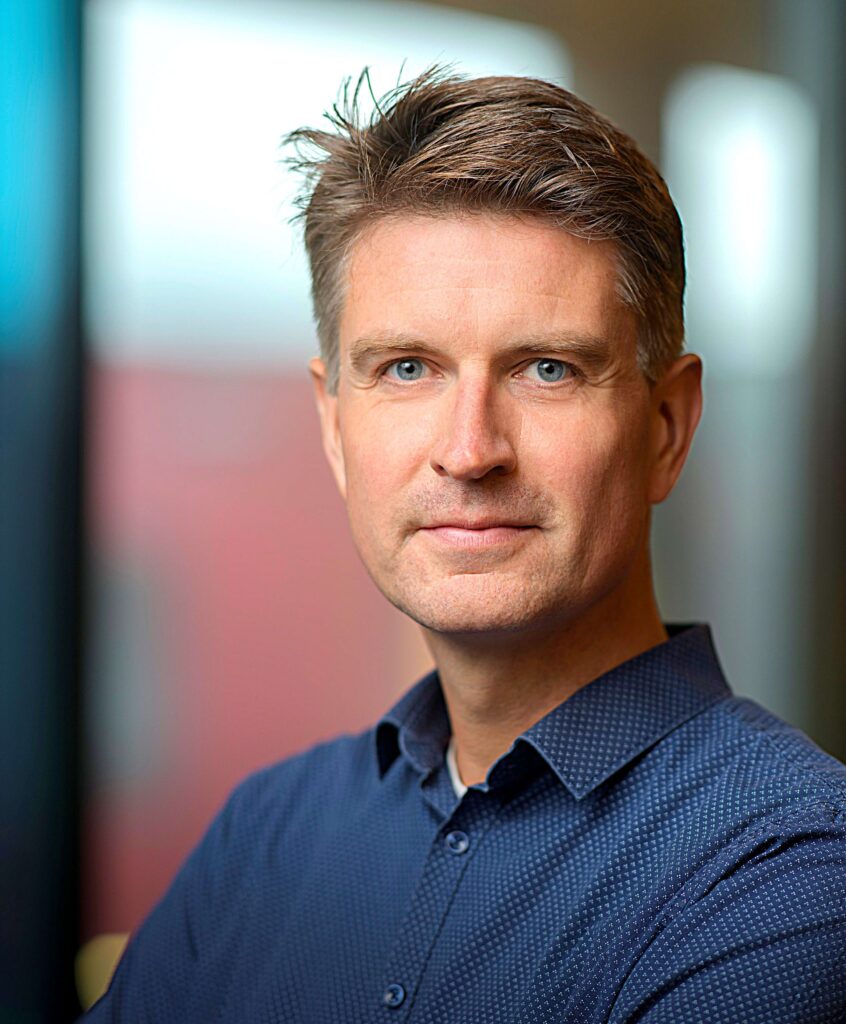
Prof.dr.ir. Wilfred van der Wiel
Chairman of the day
Prof.dr.ir. Wilfred van der Wiel is a Professor at Nano-electronics and the director of the center for brain-inspired nano systems. Wilfred van der Wiel’s research focuses on unconventional electronic devices for computing and sensing. During this year’s symposium Prof.dr.ir. Wilfred van der Wiel will guide us through the world of inorganic materials and introduce us to the speakers of the day.
The speakers for this year’s symposium are:
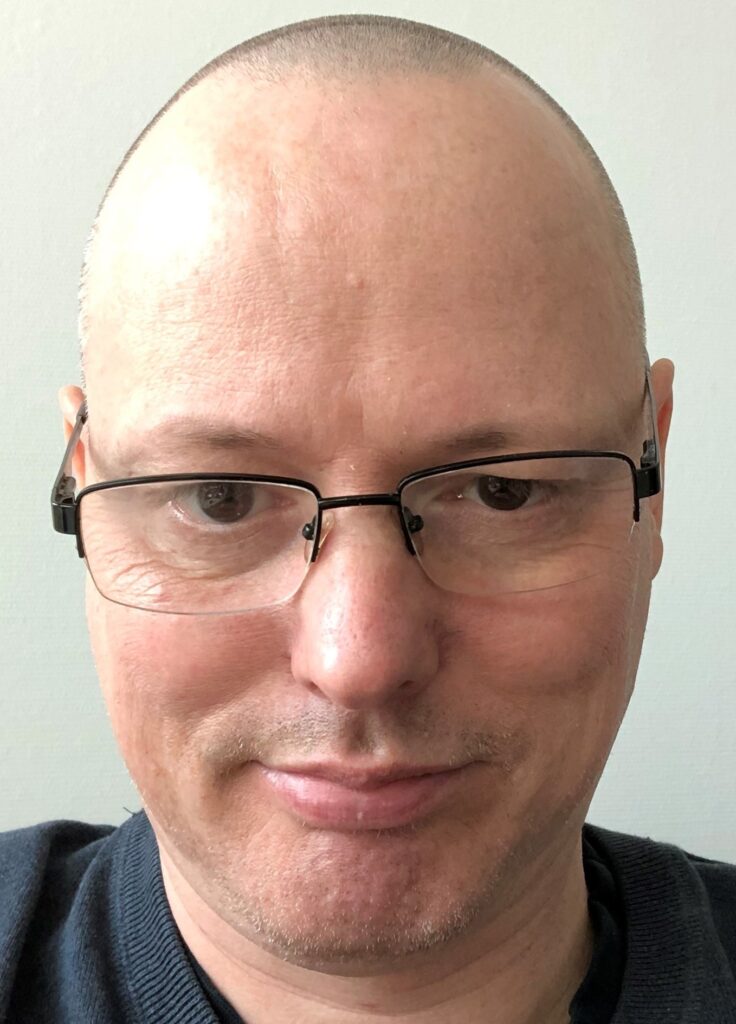
Michel de Jong
“All you ever wanted to know about band structure but were afraid to ask”.
“I will give a basic generic explanation of band structure that will hopefully provide an intuitive picture for non-experts. Starting with a comparison between classical waves and quantum mechanical waves, I will use the particle-in-a-box model and the electronic structure of simple molecules to provide a toy model for band structure. I will finish with some examples of simple solids.”
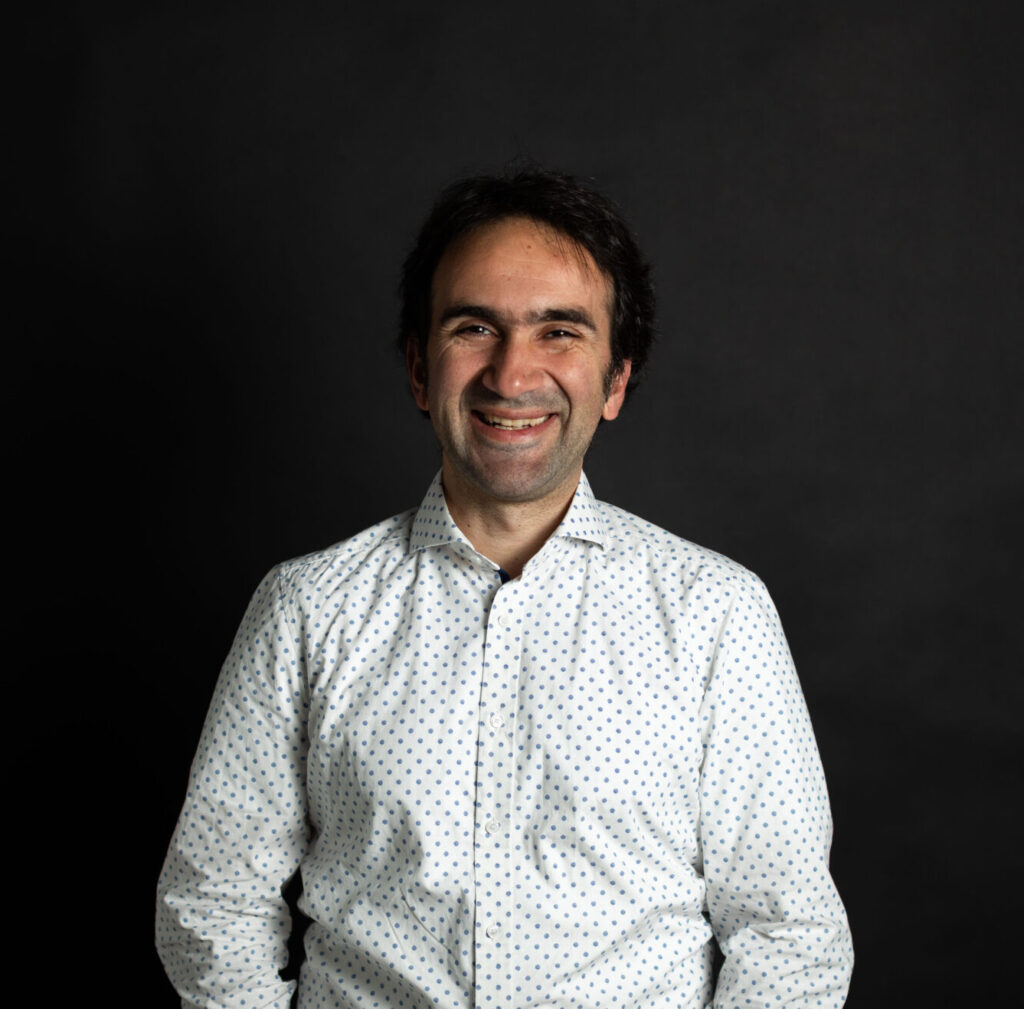
Prof. Dr. Alexander Ako Khajetoorians
“What can we “learn” from atoms?”.
There is a quest to create materials that are computers, where there’s no longer a distinction between hardware and software. Often, these concepts are routed in mimicking the basic computational properties of the brain and trying to realize them in the physical behavior of materials. Efforts toward this end requires understanding how concepts like memory and association, can be linked to fundamental physical concepts. Here, I will review the ideas linked toward this new paradigm in computing, routed in fundamentals studies based on the idea of “let the physics do the work for you.” I will then demonstrate a bottom-up platform we have developed, based on the concept of atomic orbital memory, which allows us to explore the fundamental physics related to this paradigm. In this discussion, I will review the link between multi-well energy landscapes and the dynamics of glasses. I will then show how the stochastic dynamics of coupled atoms directly mimic the perennial Boltzmann machine, the concept developed by recent Nobel Prize winner G. Hinton. I will conclude with an outlook on these concepts and future avenues in brain-inspired computing and their connections to quantum technologies.
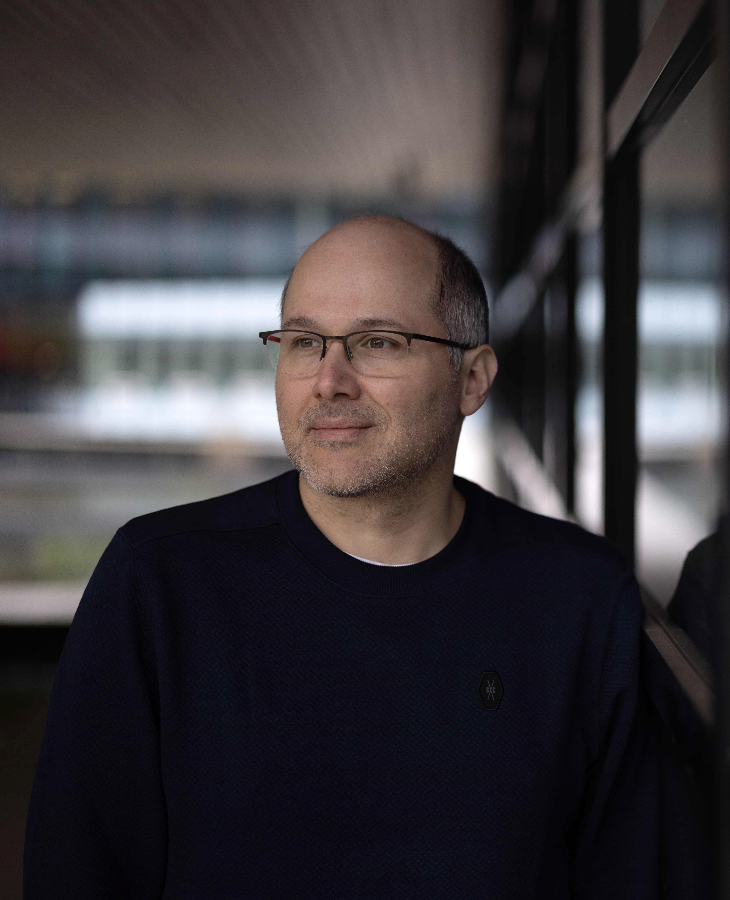
Arturo Susarrey Arce
“From Single to Multi-Material 3D Printing of Glass-Ceramics for Micro-Optics”.
3D printing, also known as additive manufacturing (AM), is a transformative technique that fabricates three-dimensional objects by depositing material layer by layer, guided by digital designs. Unlike traditional subtractive methods, AM offers key advantages, including reduced material waste, enhanced design freedom, and the ability to produce complex geometries that are difficult or impossible to achieve conventionally.
This talk focuses on the fabrication of micro- and nanometric inorganic architectures—such as glass and ceramics—using advanced AM techniques. Special attention is given to two-photon lithography (TPL), a high-resolution method that enables the creation of intricate 3D structures with sub-micron precision. TPL leverages nonlinear optical absorption to selectively polymerize materials at the focal point of a femtosecond laser, making it ideal for printing optical-grade inorganic components.
We will examine the morphological, structural, and chemical characteristics of these printed architectures, as well as their optical properties. In particular, the integration of lanthanide dopants, such as europium, is discussed. Europium serves as a luminescent probe, allowing us to track structural changes in the inorganic matrix during post-processing steps such as annealing. These insights are crucial for tailoring the functionality of printed components for sensing.
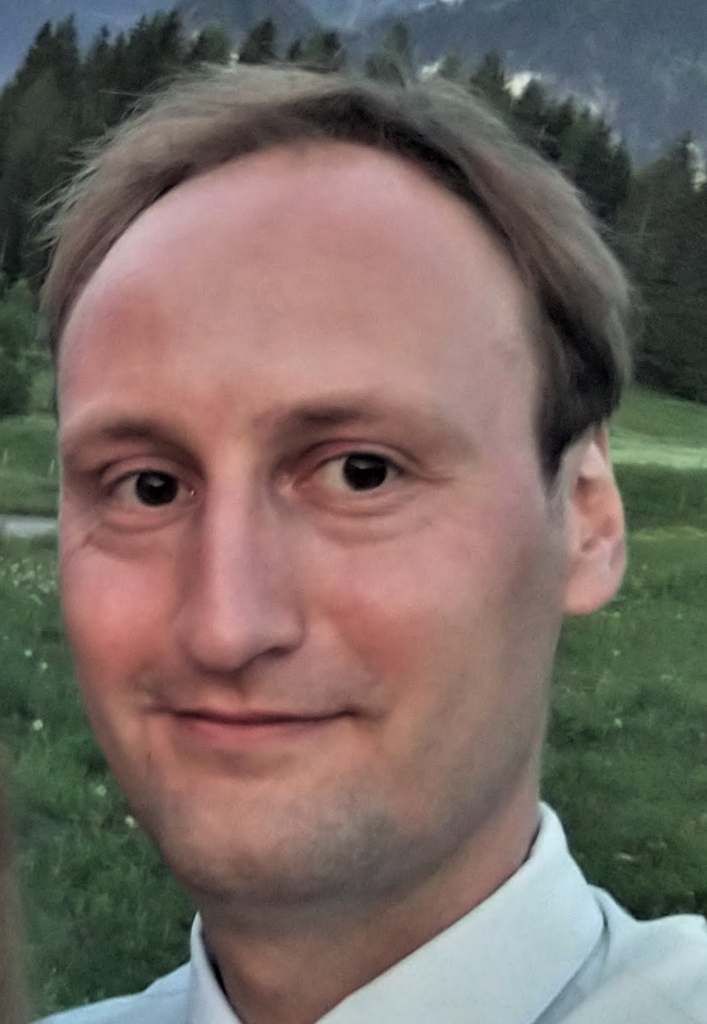
Joost Ridderbos
“The search for the fractional ac Josephson effect in topological materials”.
Ever since their discovery, topological materials have been a highly active topic in condensed matter Physics. They possess unique electrical properties, characterized by the fact that conductance can Only occur on the surface or physical boundaries, while the bulk of the material is insulating. When Reducing a topological insulator to a 1-dimensional structure and subjecting it to proximity induced Superconductivity, a unique type of topological state emerges: Localized on the endpoints of this 1- Dimensional system we now find a particle that is its own antiparticle in the form of a Majorana zero Mode. If these Majoranas can be controllably defined on a large scale, they hold promise for Constructing a fault tolerant quantum computer.
Several methods have been used in an attempt to find and characterize these peculiar particles, However no ‘smoking gun’ evidence has been found so far, and their elusiveness has led to Controversy in this particular field of research.
In our work, we aim to alleviate part of this elusiveness by attaching superconducting electrodes to Nanoscale topological materials, thus creating Josephson junctions. We subject these junctions to Voltage bias, which evokes radiation with frequency f = 2ev/h with h being Planck’s constant, V the Applied voltage bias and e the electron charge, due to the relaxation of Cooper pairs. Since Majorana Zero modes effectively only transfer single electrons, they are expected to generate a halved Frequency of f = ev/h, by which their presence can be established. In this talk I will elaborate on the Challenges and results so far on this experiment, and explain the practical implementations and Subtleties one needs to consider for successful detection of the Majorana modes.
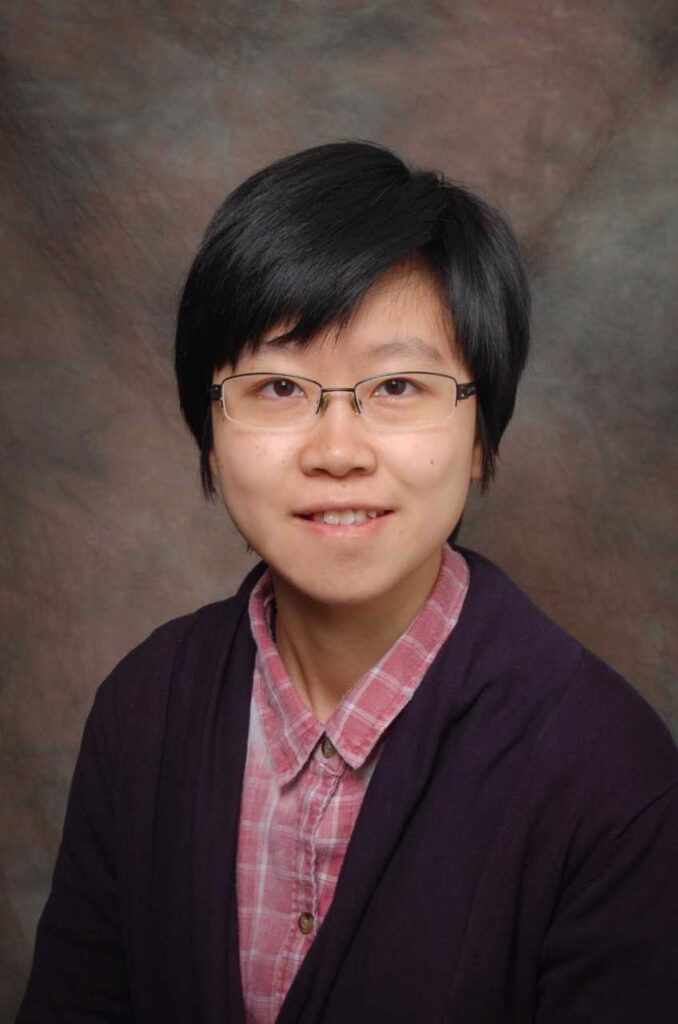
Chuan Li
“Inducing superconductivity in topological Dirac materials – detecting and creating novel states”.
The superconducting proximity effect occurs when a superconducting state is induced in a non-superconducting material by placing it in contact with a superconductor. This effect is typically manifested through Andreev bound states. It significantly expands the possibilities for realizing superconductivity in different materials and allows for the design and control of superconducting states by selecting appropriate materials.
The discovery of topological materials has been a major breakthrough in condensed matter physics. These materials exhibit robust edge or surface states that remain protected against defects and disorder. Their topological states arise from band inversion while preserving certain symmetries, leading to exotic electronic properties. Unlike conventional materials governed by the Schrödinger equation, these states obey the Dirac equation, resulting in linear dispersion. Additionally, the presence of strong spin-orbit coupling gives rise to a unique spin texture, known as spin-momentum locking, which plays a crucial role in their transport phenomena.
Combining superconductivity with topological Dirac materials can lead to novel quantum states, theoretically including topological superconductivity and Majorana bound states. The superconducting proximity effect not only makes these states possible but also serves as a highly sensitive and effective probe for their study.
In this lecture, I will introduce the fundamentals of the superconducting proximity effect and different topological materials. I will also discuss ongoing research, with a particular focus on recent advances in higher-order topological materials.
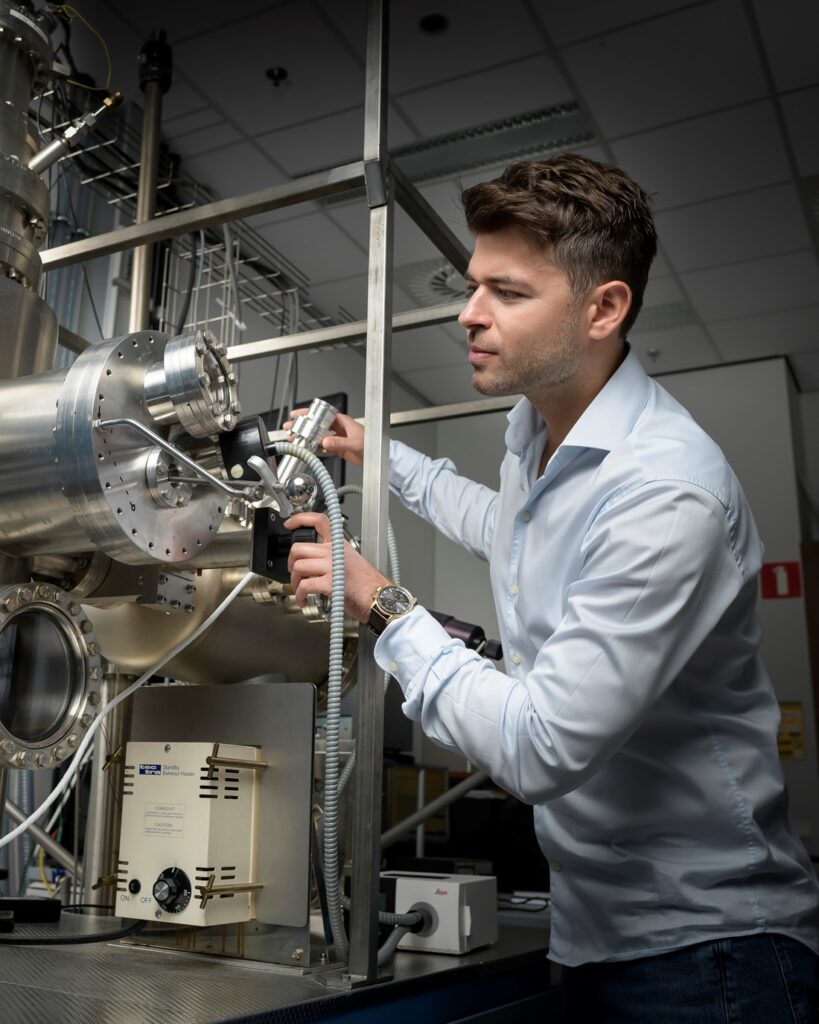
Pantelis Bampoulis
“Germanene for next-generation quantum devices”.
Two-dimensional (2D) topological insulators feature an insulating bulk and metallic edge states that are protected against backscattering by spin-momentum locking and time-reversal symmetry. These features hold promise for energy-efficient electronics and quantum computing, potentially reducing energy loss and enabling stable qubits. However, research on 2D topological insulators is hampered by their limited tunability, small bandgaps (requiring very low temperatures), limited number of topological edge states and stability issues. In Twente, we explore germanene as a 2D topological insulator that overcomes many of these challenges, offering a larger bandgap suitable for applications at room temperature and high tunability. In this talk, we will present our recent experiments on germanene, focusing on the properties of its topological states and methods to engineer these edge states for new quantum phenomena and functionalities. Finally, we will discuss future plans to integrate germanene into novel device concepts.
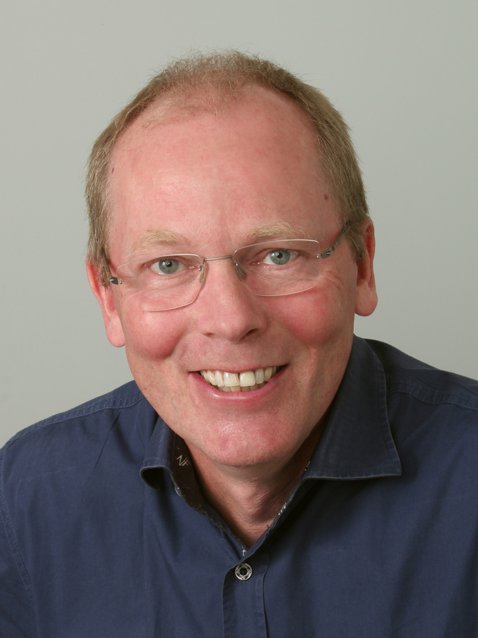
Hans Hilgenkamp
“Mind the Mott-gap”.
Besides conductors, insulators and semiconductors, a special class of materials is
formed by the Mott-materials. From their band structures, these materials should
normally speaking be electrically conducting, but due to interactions between the
conduction electrons their mobility can be reduced and the materials can even
become completely insulating.
Small changes in the materials, induced by for example chemical doping,
temperature changes or external pressure, can induce a transition from the
insulating to a metallic state.
Interestingly, such Mott insulator-to-metal transitions give rise to remarkable physical
phenomena, like high temperature superconductivity and special tunable resistance
effects.
I will introduce the Mott physics and highlight some of the research done at the
University of Twente on high temperature superconductors and on new electronic
device concepts using vanadium-dioxide VO2.